David Cronenberg’s 1999 cult classic eXistenZ imagines a world where computers are built with biological components. Characters plug into virtual reality video game consoles powered by genetically engineered amphibians. More than two decades later, scientists are starting to experiment with a similar premise — hooking mini-brains up to computers to solve biology problems too complicated for artificial intelligence algorithms alone.
Technology startups have raised billions on the promise that artificial intelligence algorithms could accelerate drug discovery, improve our understanding of the brain, and achieve “general intelligence” that surpasses human capabilities. Many think that algorithms as smart as humans are coming — half of the experts responding to a 2022 survey believed that such algorithms are only four decades away. But despite the hype, AI algorithms might be ill-equipped to resolve biology’s open questions. The first AI-designed drugs proved underwhelming in clinical trials — perhaps due to high expectations. Some experts thought AI-design would increase the clinical trial success rate from 5 or 10% to 90%, but the real impact might be substantially smaller. While some chatbots acing standardized tests seemed a sign of intelligence, critics argued that models may have been fed the answers and doubted that current algorithms will lead to a generalized intelligence because there simply isn’t enough data around.
This has prompted scientists to scan elsewhere for a boost over these limitations. They seem to have found it with small clumps of real brain tissue known as organoids.
General Intelligence in a Dish
Brain organoids are 2D and 3D cultures of brain cells that mimic the structure and properties of the brain. In the burgeoning field of organoid intelligence, researchers are interfacing these living tissues with electrodes that allow them to send and receive electrical signals.
The brain is a by-product of billions of years of evolution, providing living organisms plenty of time to fine-tune and optimize its function. As a result, when the brain goes head-to-head against AI algorithms, so to speak, it comes out on top. AI algorithms also require enormous amounts of energy to power its data centers and freshwater to cool them. The International Energy Agency projects that data centers’ electricity consumption in 2026 will hit 1,000 terawatts, roughly equivalent to Japan’s current total consumption. Meanwhile, brain cells run on “glorified sugar water,” said Brett Kagan, the chief scientific officer of Cortical Labs which grows neurons in silicon chips.
“We’re talking about hundreds of thousands or hundreds of millions of times less power,” Kagan added. It’s hard to estimate exactly how much power the brain uses, but according to an analysis done on slices of rat brains, the brain consumes about 30 watts of power each day, which is less than some lightbulbs. This tiny amount of power is sufficient to run a billion-billion calculations per second while some supercomputers require about a million times more power to match.
Brains are also much better at learning than AI models. Kagan explained that organoids can do some of these learning tasks much faster because of how neurons process information. Computers can store data in bits, which provide one state or piece of information, a one or zero. In contrast, neurons can simultaneously hold and process three different pieces of information.
Kagan also thinks that brain organoids may have the potential to develop general intelligence, or the capacity to learn and solve novel problems from memory, knowledge, reasoning, and visual-spatial processing. “We don’t know whether or not we can get silicon to actually show generalized intelligence, ” Kagan said. In contrast, “biological brain cells can lead to generalized intelligence,” so it may be possible to develop these abilities in organoids connected to computers.
For practical applications, organoids don’t need to reach human-level general intelligence. Insect-level intelligence might be good enough for solving certain problems. Bumblebees, for example, have a wide range of cognitive abilities despite only having 800,000 brain cells. In addition to navigating their environment, bees can learn complex tasks from social interactions, understand numbers, and the concept of zero.
“I know some people think it’s a controversial statement, but I think with our current technology, the time and money we could recreate bee-level intelligence,” he said, adding that these intelligent organoids could help develop accessibility devices that can help someone who is vision or hearing impaired better navigate the environment.
Teaching and Learning with Mini-Brains
We are constantly converting signals like sound, lights, and other chemicals from our environment into electrical signals. These signals reach the brain, prompting the cells to send their own electrical signals to each other as they process and perceive the information from their environment. Scientists interested in brain organoids are sending along electrical signals to mini-brains to mimic the real world experience, and are using specialized hardware to eavesdrop on the ways that mini-brains respond.
Researchers developed different types of hardware to measure and stimulate electrical activity in the organoids. Some of these approaches — like tiny EEG caps or silicon chips — interface with the cells on the organoid’s surface. Other methods involve inserting electrodes inside the organoid to target the cells inside. Different types of stimulation, such as sound, can be transduced into electrical signals and fed into the organoid. The output is fed back to a computer that can analyze the results.
In contrast to a simple cell culture, organoid models provide researchers with more data about how different drugs affect brain function, learning, and connectivity.
In 2022, neuroscientists from Cortical Labs trained a 2-dimensional brain organoid connected to a computer to play Pong. The researchers wanted to see whether the brain cells could adapt and change their electrical activity in response to input from their environment. The team created a simulated pong environment where aspects of the video game, like the location of the ball and the paddles, were transduced into electrical signals and fed to a network of 800,000 cells grown on a silicon chip.
The system didn’t just play Pong, it learned how to play better thanks to electric feedback. Everytime the cells hit the ball, they received a predictable electric burst of a specific frequency in a specific location. When the cells’ paddle missed, they received a disorganized, random burst of electrical stimulation. The researchers thought that the cells would prefer the predictable pattern of electrical activity, leading them to “learn” how to tit the ball better. It’s the type of reinforcement training we see in our own learning as well as artificial intelligence models. The network became more energy efficient over time as it learned to play the game, within five minutes.
The study sparked controversy because its authors wrote that the cells “exhibited sentience.” They argued that the cells could sense and respond to signals in their environment.
“The lack of agreement did reveal an interesting schism in the scientific and philosophical world that we took very seriously,” said Kagan, the chief scientific officer of Cortical labs. Kagan contests that the definition is accurate, according to definitions established by other researchers. “We have since been working with numerous academics across the world with the intent to work towards unifying the language across multiple intelligent systems with the intention to create clarity and improve accessibility to the public.” Since Kagan’s study was published, other researchers have pushed the limits of learning in organoids.
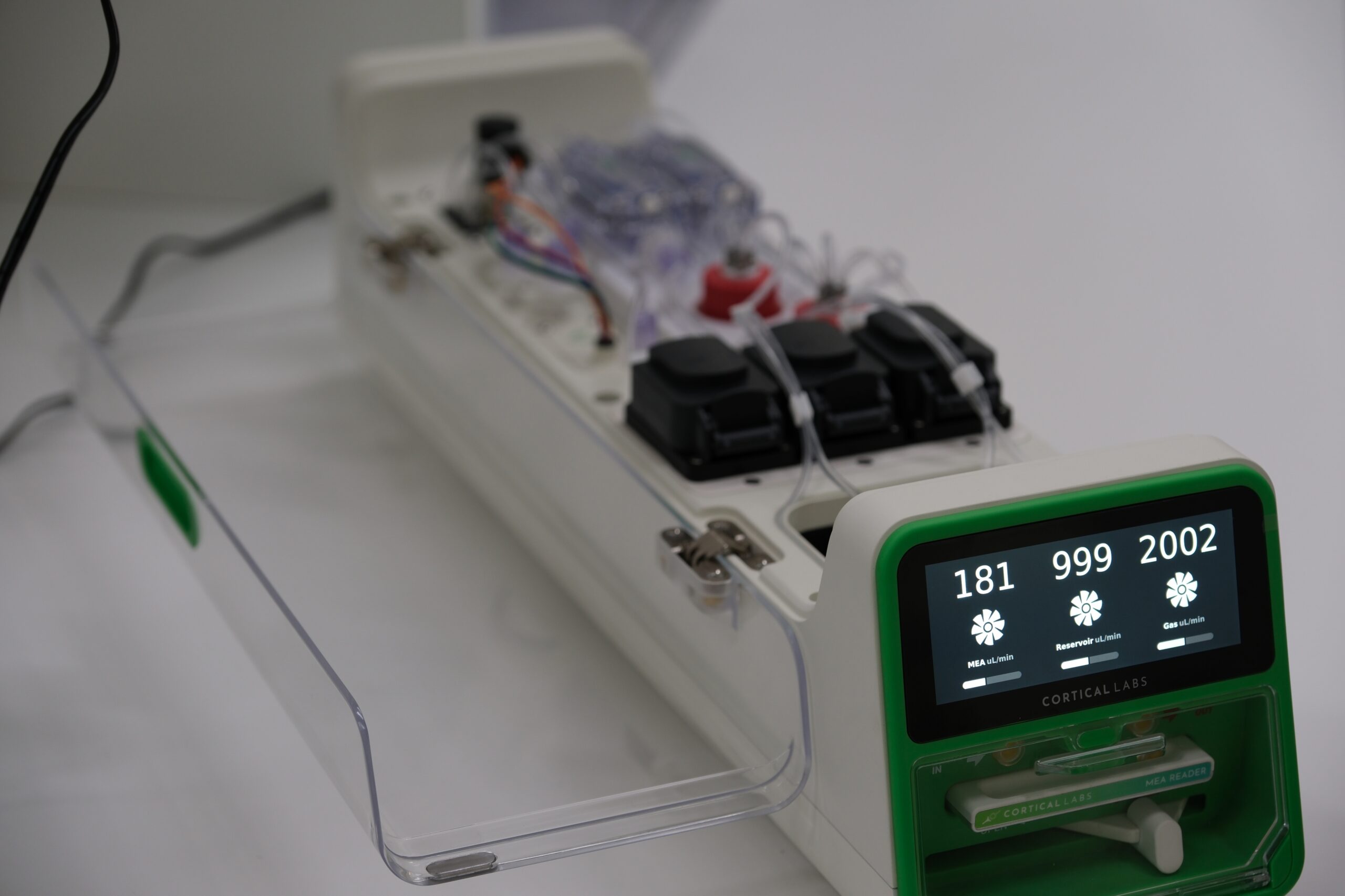
Organoid Computer Hybrids
In 2023, scientists at the University of Indiana Bloomington published a proof-of-concept study in Nature Electronics to test how well a biohybrid computer called Brainoware could recognize speech and the results could lay the groundwork for testing drugs that treat brain disorders on organoids.
Like Kagan’s team, the scientists grew the brain cells into an organoid on a silicon chip with many electrodes. The researchers transduced 240 audio clips of 8 speakers pronouncing Japanese vowels into electrical signals for an AI algorithm that uses a combination of computer hardware and the brain cells themselves to process the information. The researchers would then measure the electrical activity at the end of the process, providing a readout that would tell researchers who was speaking. The digital algorithm matched audio clips to the Japanese speaker correctly 78 percent of the time. If scientists grow organoids from the cells of people with Alzheimer’s, they could test out whether cognitive boosting medications could help the organoids perform better at speech recognition tasks. The medications that succeed in the organoid may be more likely to succeed in clinical trials than drugs first tested in mice or monkeys.
Organoids hooked up to computers also have the potential to reduce the amount of animals being used by researchers, while still delivering meaningful results. Kagan and his colleagues at Cortical Labs are already using the DishBrain model to screen different drugs to see if they affect how well their Pong-playing neurons can learn and adapt. And other scientists, like Lena Smirnova, an assistant professor at Johns Hopkins, are trying to use brain organoids to replace animal models of neurological and neurodevelopmental disease.
Since many human neurological diseases don’t occur naturally in other species, labs mimic the disease by inserting human-like genetic defects into mice and rats. However, some of these disease models, most notably for Alzheimer’s, have proven to be ineffective analogs of the human brain. As a result, by 2017 more than 99.6 percent of drugs that make it to human testing for Alzheimer’s have failed. Since then, aducanumab which received a partial approval from the Food and Drug Administration (FDA) has been taken off the market while two other drugs that have since been approved are controversial among neurologists over whether or not the benefits outweigh the risks. (One of these drugs failed to receive approval in Europe). Brain organoids developed from human cells may paint a more accurate picture.
Researchers can use a chemical cocktail to revert blood or skin cells into pluripotent stem cells. Then, over the course of 35 to 45 days, scientists can add chemical cues to differentiate and mature the induced pluripotent stem cells into a 2-D or 3-D culture of brain cells.
Smirnova’s lab is moving toward making these models more complex and accurate by adding more glial cells — supportive cells in the brain — to the organoids. There are as many glial cells as there are neurons in the brain. For certain experiments, researchers can also edit in reporter genes that could provide even more information. Reporter genes might activate, for example, when the cells in the organoid activate a pathway involved in memory, causing the cell to make a fluorescent protein. This could be useful in a model of Alzheimer’s, where memory pathways are impaired. Researchers could see if drugs would improve performance on a memory task, and can check to see that the drug is working by measuring the levels of the reporter.
“There’s very strong arguments to say that it’s more ethical to use an induced pluripotent stem cell than it is to kill animals,” Kagan said. Typically, companies may need to test approved drugs in animal models to test whether they work for another disease but advanced organoid models may make it possible to skip this step altogether. But the tide is changing.
In 2021, the FDA authorized Sanofi to run a clinical trial to repurpose one of their drugs for a rare neuromuscular disease based on data obtained from an organ-on-a-chip model. The researchers who tested the organ-on-a-chip model hope that these approaches can accelerate the path to clinical trials. Sanofi’s clinical trial is currently testing the drug in 110 participants, with results expected by the end of 2025.
The Ethics of “Teaching” Mini-Brains
Using organoids to run experiments on memory and learning, and to make biohybrid computers raises many ethical concerns. Researchers are working closely with ethicists to identify and address these issues. The ethics team working with Smirnova and her colleagues has developed surveys to help researchers understand public concerns and perspectives on using their cells in experiments involving learning and memory, providing researchers with feedback from diverse moral perspectives.
While some have voiced concerns of organoids gaining consciousness, Smirnova isn’t one of them: “I don’t expect any consciousness from just cells which are connected to each other,” she said. “But we need to be ready.”
The more familiar ethical concern deals with confidentiality: Must researchers tell donors if they discover something relevant about their health, such as a genetic variant that confers a high risk of developing a disease in the future which the researchers might spot in the course of studying their organoids? And do those donors own a stake in the data or technology derived from their cells? Despite the potential for commercial and medical breakthroughs, these questions remain unanswered.
Still, the research plows forward. Organoids hooked up to electrodes are already being used to improve our understanding of the brain and to repurpose or test new drugs. In the future, Kagan thinks that organoid intelligence could give rise to another computational tool — a biohybrid computer.
Cortical Labs is now developing devices that make it easier to set up closed-loop brain organoid systems, like in their Pong experiment, where cells can receive and respond to various stimuli in real time. “The idea is to make it easy and accessible for anyone to create more complicated virtual environments for neural systems to be embodied in with bespoke hardware and software tools.”